Recent News
CHTM Joins NSF's NQVL Pilot Projects
August 9, 2024
OSE PHD, Dr. Xuefeng Li - Wins The Outstanding Interdisciplinary Graduate Programs Award
May 10, 2024
Dr. Ali Rastegari - 2024 OSE Best Dissertation Award Winner
May 10, 2024
2024 OSE Spring and Summer Graduates
May 10, 2024
News Archives
Recent discoveries shrink the observed scale of time and space
November 30, 2016 - Compiled by Sharon Steely for CHTM
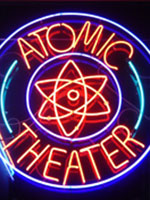
Time
Attosecond streaking spectroscopy used to observe the breakdown of a single active-electron
When light strikes electrons, they get excited and can break free from their atoms. The photon’s energy is either entirely consumed by one electron or divided among several. This electron ejection is known as the photoelectric effect. 1
Albert Einstein described this tricky quirk of light in 1905, later winning the Nobel Prize in Physics for his explanation of this defining concept. The photoelectric effect shows that light can act as both a wave and a particle. When a photon, or a particle of light, of a certain energy strikes an electron, it can free the electron from its atom. The photon ejects the electron in a process called photoemission, the basis behind solar energy. 2
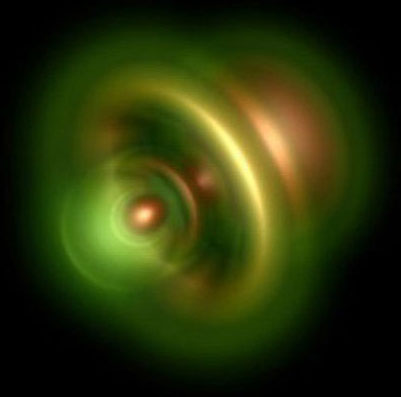
Electron escaping a helium atom (M. Osslander (TUM) / M. Schultz (MPQ))
Image republished from New Scientist
Previous experiments studying this effect could only measure what happened after the electron was kicked out of an atom, says Martin Schultze at the Max Planck Institute of Quantum Optics in Garching, Germany. 1 Now, he and his colleagues have measured the entire ejection of electrons from a helium atom from start to finish with zeptosecond precision (10-21 seconds), marking the smallest time slot ever measured. 1
In a series of experiments, the team fired an unspeakably brief, extremely ultraviolet laser pulse at a helium atom to start exciting its pair of electrons. This pulse lasted just 100 to 200 attoseconds, or 10-18 seconds. But by making many readings and calculating their statistical spread, they were able to measure events at a rate of 850 zeptoseconds. 1
A zeptosecond is a trillionth of a billionth of a second.
“Using this information, we can measure the time it takes the electron to change its quantum state from the very constricted, bound state around the atom to the free state,” says Marcus Ossiander at the Max Planck Institute. 1
One reason for choosing helium is because it has just two electrons, allowing direct measurement of their quantum mechanical behavior. For an atom with more electrons, some assumptions would be needed about how the energy was divided and the time taken for ejection. 1
The results are an important window into the quantum behavior of atoms, especially how their electrons work, Schultze says. Understanding that could lead to insights into phenomena like superconductivity or quantum computing. 1
“There is always more than one electron. They always interact. They will always feel each other, even at great distances,” he says. “Many things are rooted in the interactions of individual electrons, but we handle them as a collective thing. If you really want to develop a microscopic understanding of atoms, on the most basic level, you need to understand how electrons deal with each other.” 1
From the Abstract:
Photoemission of an electron is commonly treated as a one-particle phenomenon. With attosecond streaking spectroscopy we observe the breakdown of this single active-electron approximation by recording up to six attoseconds retardation of the dislodged photoelectron due to electronic correlations. We recorded the photon-energy-dependent emission timing of electrons, released from the helium ground state by an extreme-ultraviolet photon, either leaving the ion in its ground state or exciting it into a shake-up state. We identify an optical field-driven d.c. Stark shift of charge-asymmetric ionic states formed after the entangled photoemission as a key contribution to the observed correlation time shift. These findings enable a complete wavepacket reconstruction and are universal for all polarized initial and final states. Sub-attosecond agreement with quantum mechanical ab initio modelling allows us to determine the absolute zero of time in the photoelectric effect to a precision better than 1/25th of the atomic unit of time.
Research
The research was published in November 2016 in Nature Physics: DOI: 10.1038/nphys3941
Sources:
- The above post was excerpted from an article by by Rebecca Boyle, New Scientist.com: Smallest sliver of time yet measured sees electrons fleeing atom
- Additional information was included from an article by Jason Daley, Smithsonian.com: Meet the Zeptosecond, the Smallest Slice of Time Yet Recorded
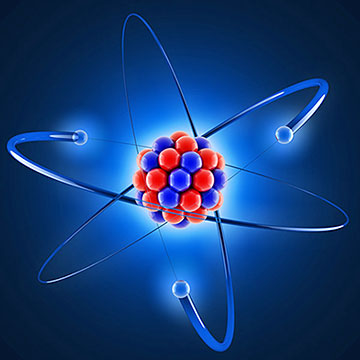
Space
Single-molecule optomechanics in “pico-cavities”
Material scientists and nanophotonics researchers from Cambridge University and the Centre for Materials Physics in San Sebastian, Spain, have developed a “magnifying glass” that can focus light down to the scale of single atoms.
The invention has the potential to unlock light-catalysed chemical reactions and to be used in opto-mechanical data storage devices.
Previously, it had been thought that it was not possible to focus light into a spot smaller than its wavelength. The Cambridge-led team used conductive gold nanoparticles to create an optical cavity so small that a single molecule could fit inside it. Known as a “pico-cavity” by the researchers, it consists of bump in a gold nano structure the size of a single atom.
This “pico-cavity” confines light to a space less than a billionth of a meter across.
Constructing the pico-cavity involved building a structure with single atom control. The Cambridge team sandwiched a layer of a self-assembling organic molecule, biphenyl-4-thiol, between a film of gold and a gold nanoparticle, with the whole assembly cooled to -260°C to reduce the speed of the atoms’ motion, and used lasers to move individual atoms in the nanoparticle, and allowed them to observe the atoms’ motion in real-time.
“Even single gold atoms behave just like tiny metallic ball-bearings in our experiments, with conducting electrons roaming around, which is very different from the quantum life where electrons are bound to the nucleus,” said Prof Jeremy Baumberg of the Nanophotonics Centre at Cambridge’s Cavendish Laboratory, who directed the project. Theoretician Prof Javier Aispurua of San Sebastian added: “Our models suggested that individual items sticking out might act as tiny lightning rods, but focusing light instead of electricity.”
The published report describes the process:
Confining light to a cavity is often used to enhance the interaction between the light and a particle stored within the cavity. Benz et al. worked with a self-assembled monolayer of biphenyl-4-thiol molecules sandwiched between a gold film and a gold nanoparticle. They used laser irradiation to move atoms in the nanoparticle and produced a “picocavity” that was stable at cryogenic temperatures. The authors were then able to obtain time-dependent Raman spectra from individual molecules. Such subwavelength cavities that can localize light to volumes well below 1 nm3 will enable optical experiments on the atomic scale.
Research
The research was published in November 2016 in the journal Science, DOI: 10.1126/science.aah5243
Source:
The above post was reproduced from an article by Stuart Nathan in The Engineer, ‘Picocavity’ focuses light below atomic scale